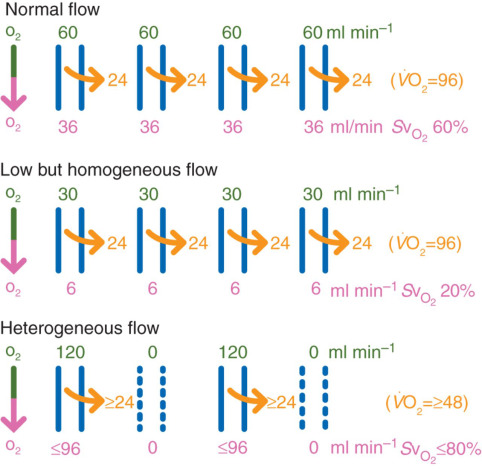
Microcirculatory dysfunction and resuscitation: why, when, and how
J.P.R. Moore, A. Dyson, M. Singer, J. Fraser – Open Archive DOI:https://doi.org/10.1093/bja/aev163
The study delves into the complex world of the microcirculation and its endothelium, emphasizing their pivotal role as the body’s largest organ. The microcirculation consists of vessels smaller than 100–150 µm in diameter, including arterioles, venules, and capillaries. It highlights the intricate branching pattern of arterioles and discusses the unique microcirculatory architecture found in organs like the kidney, gut, and liver due to their specific functional requirements.
Perfusion of the microcirculation is regulated by myogenic, metabolic, and neurohumoral mechanisms that control blood flow at a local level. Myogenic autoregulation allows blood vessels to constrict or dilate in response to changes in pressure, while metabolic regulation ensures matching of local blood flow to tissue metabolic needs. Neurohumoral modulation further influences arteriolar tone and capillary flow distribution within functional units.
The study also explores microcirculatory dysfunction in various types of shock, including hypovolaemic shock, cardiogenic shock, and septic shock. In hypovolaemic shock, sympathetic responses vary within different tissues, leading to vasoconstriction in some areas and dilation in others. Cardiogenic shock involves reduced microvascular perfusion due to altered vascular tone, rheological changes, and inflammatory responses. In septic shock, a hyperdynamic vasodilatory state and vascular autoregulatory dysfunction contribute to heterogeneous capillary perfusion and oxygen supply deficiency.
Overall, the study sheds light on the intricate mechanisms governing microcirculatory function and dysfunction in different pathological conditions, highlighting the importance of understanding these processes for improving patient outcomes.
Impact of heterogeneous perfusion on tissue metabolism and venous oxygen saturation ( 𝑆vO2). In the normal situation (top panel), O2 is delivered at 240 ml min−1 in four perfused capillaries. The tissues extract oxygen to meet cellular oxygen consumption ( 𝑉˙O2). In the presence of low-flow but homogeneous perfusion (middle panel), half the O2 is delivered to the tissue but all the capillaries are perfused. The amount of oxygen is sufficient to meet the oxygen requirement of the cells; hence, 𝑉˙O2 is preserved even though 𝑆vO2 is severely decreased. In the presence of heterogeneous flow (bottom panel), even though total oxygen delivery is preserved (240 ml min−1), only 50% of the capillaries are perfused. Cells close to the perfused capillaries consume the normal amount of oxygen. Cells too far away from perfused capillaries do not receive enough O2 to meet their O2 requirements and become hypoxic. It is possible that the proportion of O2 extracted from perfused capillaries increases in order to compensate for those which are poorly perfused. The degree of any compensation is uncertain, but it is unlikely to restore normal oxygen delivery to the vascular bed as a whole. As a consequence, hypoxic zones can be encountered in the presence of an elevated 𝑆vO2. Note that this schematic presentation is simplified. In normal conditions, recruitment of the microcirculation is not maximal, and a mild degree of heterogeneity can be observed. In response to systemic low flow, such as illustrated in the middle panel, the microcirculation tends to adapt by recruiting previously unfilled capillaries and decreasing perfusion heterogeneity. When endothelial dysfunction occurs and heterogeneity develops, such as in the bottom panel, these adaptive mechanisms are lost. (Adapted from De Backer and colleagues, with permission of Springer Science+Business Media.)
The microcirculation plays a crucial role in delivering oxygen to tissues. In normal conditions, it ensures that tissues receive enough oxygen, even if there are changes in overall blood pressure or heart function. However, in diseases, the microcirculation can stop working properly, leading to shock. If the microcirculation can be quickly restored, outcomes can be improved. Monitoring the microcirculation during treatment could be better than just looking at overall blood flow. This might lead to more careful treatment, especially considering the potential harms of some treatments. In advanced cases of shock or organ failure, the role of microcirculation monitoring is less clear.
The body’s priorities may shift to protecting organs, and the microcirculation might adapt to limit further damage. The level of microcirculation restoration needed could vary depending on the type and stage of illness. Current methods for monitoring blood flow are not very good at detecting problems at the tissue level. Technologies to monitor the microcirculation at the bedside are still being developed, and there’s no agreement on what goals to aim for in microcirculation-focused treatment.
Read the full study: https://www.bjanaesthesia.org/article/S0007-0912(17)31149-2/fulltext